Energy use and its impact on the environment are among the most important technical, social and public policy issues that face humankind today. The debate about whether climate change is real has gone on too long and the time has come to develop practical solutions. This month world leaders will gather in Copenhagen to try to formulate a new international climate change treaty to replace the failed Kyoto agreement. Whatever the result of these deliberations, the world knows that urgent action is required in order to reduce greenhouse gas emissions if we are to keep global temperature increases within manageable limits. Even if strict greenhouse gas targets are agreed upon in Copenhagen by the world community, without strong leadership to develop new and imaginative policies in each country the agreement is doomed to failure, just like Kyoto. In this essay I try to outline some of the real world solutions that can be used to lead us to a more sustainable future.
With 80 percent of current energy supplies from fossil fuels, we have been stuck in the carbon economy for a long time. In broad terms there are only four ways to reduce the greenhouse gas emissions resulting from our over-reliance on fossil fuels. Simply put, these are the following: using less energy, using energy more efficiently, employing carbon capture and storage techniques, and switching from fossil fuels to other sources of energy. Choosing to use less energy just means that each one of us can reduce our impact on the climate through activities such as walking or cycling rather than driving a car, or by turning down the thermostat in our houses and offices. Over a longer time period we can also make our communities more energy efficient using design guidelines and policies that increase housing density and encourage employees to live close to their workplace. These both require changing the attitudes and habits of a large majority of the population, a daunting task. Just as with the recycling revolution, these lifestyle changes are likely to be led by young people setting an example for the rest of us. Once a tipping point has been reached, it will become socially unacceptable not to be seen to be doing something to reduce our individual climate footprint. Governments need to recognize the power of engaging their citizens in this way, and should establish education and information programs in order to expedite the transition to a lower energy lifestyle. So far very little of this communications work has been done in Canada compared to European countries.
The second approach to reducing fossil fuel consumption, and therefore reducing our carbon dioxide emissions, will rely on a dedicated effort to increase energy efficiency. This will reduce the demand for energy in industrial processes, in consumer products such as cars and appliances, and in the heating and cooling systems for commercial and residential buildings. Although the energy efficiency of buildings and industrial processes has been increasing over many years, there is still a long way to go before we can say that we are not needlessly wasting energy and producing more greenhouse gas emissions than we need to. Increasing energy efficiency is often seen as the low-hanging fruit in plans to reduce fossil fuel consumption, and can often result in significant economic benefits to industry and consumers alike.
There is very little new technology required to pursue energy efficiency aggressively, since most techniques are already well established. These include increasing building insulation, replacing single-glazed windows with double-glazed units and replacing old furnaces with new high-efficiency condensing units. There are often financial barriers, however, since in most cases there is a considerable up-front capital cost, while the savings accrue over time from the reduced cost of energy. Institutional barriers can also be important, with the best example being the landlord-tenant relationship in which a tenant pays heating costs while the landlord pays the capital cost for any improvements to heating and cooling equipment. In this case, there is no incentive for the landlord to make improvements, while the tenants are powerless to take any action to increase the efficiency of their energy use and reduce their costs. Many of these situations can be remedied by government action through the provision of low-cost loans for capital improvements, as well as regulations to ensure that landlords are encouraged to make their buildings as energy efficient as possible. Once again, in terms of both financial support and regulations, Canada lags far behind other developed countries at this time.
The third approach to reducing our ever-increasing greenhouse gas emissions is to make use of carbon capture and storage techniques. These would be applied primarily to large stationary consumers of fossil fuels, such as coal- and gas-fired power plants. Other large emitters of carbon dioxide suitable for CCS include industrial plants such as cement kilns. There are several techniques that are well established for separating carbon dioxide from exhaust gases, but the very large gas volumes involved necessarily require large and expensive equipment. The more difficult concept to evaluate, however, is the effectiveness and safety of the storage reservoirs that will be required to store carbon dioxide for thousands of years. These include depleted oil and gas reservoirs, as well as underground saline aquifers and unminable coal seams. There is much enthusiasm for these techniques from major producers of fossil fuels, such as the large oil companies, and from major coal-producing regions of the world, including the province of Alberta. However, although there are several ongoing demonstration projects underway, including one in the province of Saskatchewan as well as one in Norway, there is still a long way to go before this can be considered an economic and secure long-term solution to carbon dioxide emissions. And, of course, this will not be an option for mobile consumers of fossil fuels, such as motor vehicles, which consume most of the world’s oil production.
The fourth option to combat climate change, and the main focus of this essay, relies on “fuel-switching” away from fossil fuels to more sustainable primary energy sources, such as renewable energy and nuclear power. Although there is a great deal of discussion in the popular press about moving to a more sustainable energy supply, most commentators take only a partial view of the consequences of doing so, without regard to the overall effect on the environment. It is important to realize that whenever we use energy, whether it is to heat our homes or power our cars, we are converting one form of energy into another, or into useful work. To heat buildings, for example, the chemical energy in natural gas or fuel oil is converted into heat by the furnace. And, when we drive our cars, the engine converts the chemical energy in the gasoline into mechanical work. These are just two examples of the energy conversion chain that is always at work when we use energy in our homes, offices and factories, or on the road. Not only does the chain track the complete process from primary energy source to final end use, but it also demonstrates how much energy is lost along the way, an important factor to consider. This process can best be visualized in the accompanying diagram:
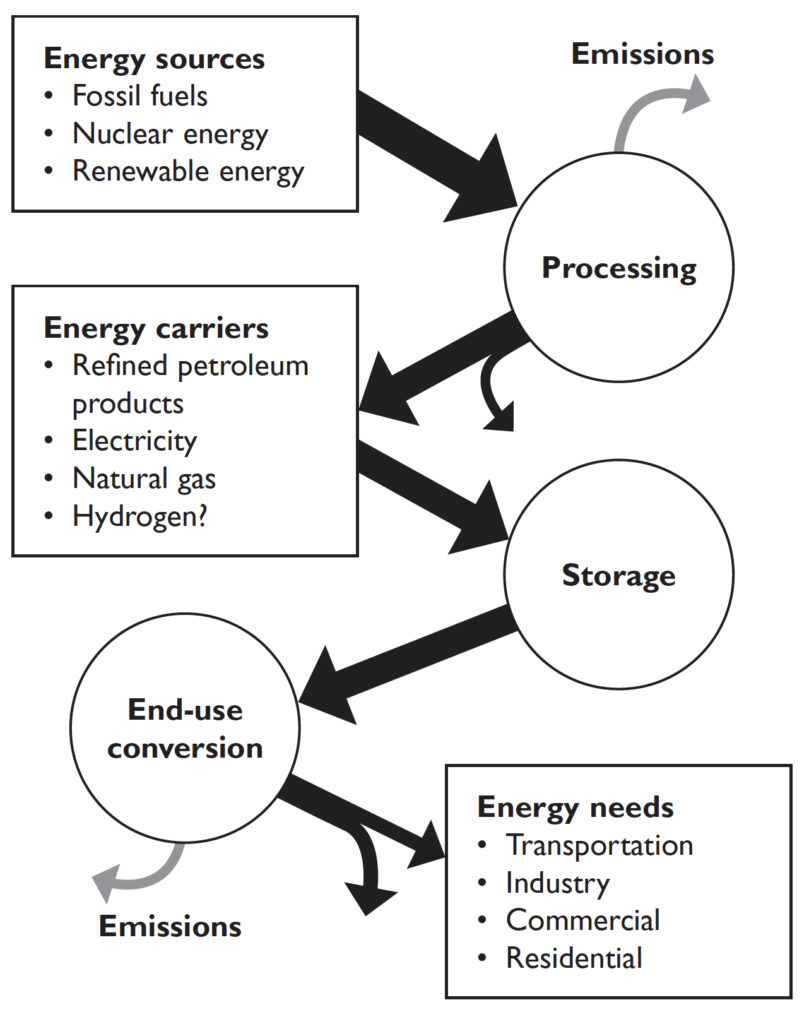
The chain starts with three primary energy sources and ends with applications to building heating and cooling, transportation and industrial processes. In between the primary source and the ultimate end use are a number of steps in which the primary source is converted into an energy carrier or is stored for use at a later time. To take a familiar example, in order to drive our cars, we use a fossil fuel—crude oil—as the primary energy source. The crude oil is first converted in a refinery into gasoline, which is the energy carrier for this case, with some loss of available energy, as indicated by the branched arrow joining the processing block to the energy carrier block. The gasoline is then stored in a fuel tank, ready for use by the engine in the final end-use conversion step, again with losses of the available energy as indicated by the branched arrow. This is just one example, but any use of energy can be tracked through the energy conversion chain in this way. One important lesson to be taken from the schematic is that there are only three primary sources of energy: fossil fuels, nuclear energy and renewable energy. And there are only three energy carriers that are significant today: refined petroleum products, natural gas and electricity. Hydrogen, often billed erroneously in the popular press as an energy source of the future, is only a potential energy carrier.
Transportation accounts for just over a quarter of the global demand for energy and provides an ideal example of the use of the energy conversion chain for analysis. Liquid petroleum fuels are ideally suited to transportation applications because of their inherently high energy density and ease of transportation and storage. The problems with using fossil fuels, of course, are that they are a non-renewable resource and that, following combustion, all of the carbon in the fuel is converted into carbon dioxide, the principal greenhouse gas. The search is continuing, therefore, to find alternative energy sources for transportation, so that the very large contribution to greenhouse gas emissions from this sector can be minimized.
Proponents of the hydrogen economy claim that the use of hydrogen as a transportation fuel would eliminate the production of any harmful exhaust emissions from vehicles on the road. This is true for the vehicle itself, but if we consider the complete energy conversion chain, since hydrogen is just an energy carrier, it would need to be manufactured from one of the three primary energy sources. If produced from hydrocarbons, such as natural gas or coal, all of the carbon in the primary energy source would still end up as carbon dioxide at the point of hydrogen production. If, on the other hand, the hydrogen was produced from a more sustainable primary source, such as from electricity generated by renewable energy or nuclear power, then there would indeed be no production of greenhouse gases. However, there is a loss of available energy at every step in the conversion chain, so that the overall energy efficiency using hydrogen is around 35 percent. In other words, only about one third of the primary energy is actually being used to drive the vehicle. A battery electric vehicle takes a much simpler approach, with a battery used on board the vehicle to store the electricity directly. In this case, the charging and discharging process of the battery has an efficiency of nearly 90 percent, so that the overall energy conversion process would only consume about one third the primary energy required for a hydrogen-fuelled vehicle. This is why most car manufacturers are now pushing very hard to develop electric vehicles, and why interest in hydrogen as an energy carrier for vehicles has subsided dramatically.
Unfortunately, batteries are not yet able to compete with liquid fuels in terms of energy density, which is a measure of the amount of energy contained in a given mass or volume. This low energy density greatly reduces the vehicle’s range before recharging is needed. Pure battery electric vehicles, such as golf carts, will likely be suitable only in specialized short-range applications for the foreseeable future. However, much of the recent development work on batteries has been driven by the introduction in the last few years of hybrid electric vehicles that use a combination of electric batteries and gasoline. Compact hybrids, the Toyota Prius being the best-known example, have been received very well by the public, and the technology is now spreading to larger cars and sport utility vehicles where the benefit of much greater fuel economy is particularly welcome. Hybrid vehicles currently on the market are classified as “stand-alone” or “grid-independent” hybrids. This means they obtain all of their primary energy from the fuel carried on board the vehicle and do not need to be plugged into the electrical grid to recharge the battery. With the expected advances in battery energy density, these vehicles have set the stage for a transition to the next generation of vehicles, the so-called “grid-connected” or “plug-in” hybrids. In these vehicles the battery pack will be much larger and can be fully charged when not in use by being plugged in at home or while at work. The engine, however, will be smaller and will still operate on some form of liquid fuel. In this way the vehicle will have a limited range of perhaps 100 kilometres as a completely electric vehicle. Beyond this distance the engine, running on gasoline or diesel fuel, will recharge the battery to extend the all-electric driving range. Recent studies have shown that a plug-in hybrid vehicle with a 90-kilometre all-electric range could provide up to an 85 percent reduction in carbon dioxide emissions for most commuters if the electricity is generated from non-fossil sources.
Switching from gasoline to electricity as the main vehicle fuel will provide the potential for a large reduction in greenhouse gas emissions, depending on the energy source used for electricity generation. In many parts of the world electricity is generated from coal and this will just have the effect of shifting carbon dioxide emissions from the vehicle to the electricity supply. There will still be a net reduction in greenhouse gas emissions, however, since electricity generation and the electric drivetrain are much more efficient than the internal combustion engine. Electrical utilities will also benefit from more electric vehicles on the road since most will be charged overnight. The increased electrical load, which is normally low at these times, would ensure that electrical generation capacity is better utilized, with the result being increased efficiency and a reduction in electricity generation costs. Ultimately, if most electricity is generated primarily by sustainable primary energy sources, such as renewable energy or nuclear power, then road transportation will no longer be a significant factor in contributing to greenhouse gas production. This will usher in a new electricity economy and mark the transition from an oil-dominated economy to one substantially based on sustainable electricity generation.
A shift from petroleum products to electricity as the transportation energy carrier of choice will also be a pleasant surprise for drivers, at least in Canada where we have some of the lowest electricity prices in the world. In many large Canadian cities, for example, the residential electricity rate is between 6 cents and 8 cents per kilowatt. On a straight energy basis this equates to an equivalent energy cost of approximately 70 cents per litre of gasoline. However, an all-electric vehicle drivetrain has an efficiency at least double that of a gasoline-powered vehicle. The effective cost of “filling up” with electricity will therefore be the equivalent of about 35 cents per litre of gasoline. These figures do not include road taxes, however, which will eventually have to be shifted to electricity once electric vehicle market penetration becomes significant. Current gasoline prices in my home province of British Columbia, for example, include a federal excise tax of 10¢ per litre and a provincial tax of 14.5¢ per litre. Even if these road taxes are eventually transferred to electricity, the price of filling up with electricity will still be a real bargain: consumers will soon realize that they can reduce their environmental footprint while, at the same time, significantly decrease the cost of transportation by switching from gasoline and diesel fuel to electricity. This will no doubt lead to most drivers enthusiastically embracing the new technology.
In the longer term, a transition to the new electricity economy will also likely include a shift from fossil fuels to electricity for heating our homes and commercial buildings. The direct use of electric resistance heating—for example in baseboard heaters—is usually seen as a poor use of electricity, particularly if the electricity is generated from fossil fuels. In this case, the overall efficiency of the energy conversion chain is much lower than if the fossil fuel, usually natural gas, is used directly to provide space heat. However, if we move to greater use of renewable and nuclear sources of electricity, the use of heat pumps for space heating becomes a much more attractive alternative.
A heat pump, working like a refrigerator in reverse, takes in energy from the air or the ground at a relatively low temperature and then delivers it at a higher temperature to provide building space heating. Electrical energy is still required to drive the heat pump, of course, but because it simply pumps heat from a lower temperature to a higher one, it delivers heat equivalent to two to three times the electrical energy consumed. The capital cost of the heat pump system is much higher than a simple resistance heating system, but as energy costs increase this capital cost can usually be offset by reduced operating costs. An added benefit of a heat pump system for building heating is that the heat pump can be run in reverse during the summer, and can therefore provide both summer air conditioning and winter heating. This feature can make heat pumps a very attractive choice in regions such as central Canada with large temperature changes from winter to summer. In these colder parts of the country, ground-source (or geoexchange) heat pumps that access heat stored underground generally work best. Ground temperature below the frost line remains quite constant at approximately 10 degrees Celsius.
In milder climates, such as on the west and east coasts of Canada, air-source heat pumps can be very effective in providing space heat at very high efficiency. In these systems heat is obtained from the outside air using a heat exchanger mounted near the house that is then pumped to a higher temperature for use inside the house. Although the capital cost of heat pumps is significantly higher than either electric baseboard heating or a gas furnace, the much higher energy efficiency enables the capital cost to be recovered in just a few years through electricity savings.
Once significant numbers of plug-in hybrid vehicles and heat pumps enter the market, shifting energy demand from fossil fuels to electricity, there will be a need to expand electrical generation capacity significantly. The impact on electrical demand may be less than some observers have predicted, however, due to the overall increase in energy efficiency of electrical systems. A recent study in the United Kingdom found that a 10 percent market penetration of electrical vehicles would only increase the demand for electricity by 2 percent. Since it will take some time to reach such levels of market penetration, utilities will have enough time to plan for an orderly expansion of their generation capacity to accommodate the shift to the electricity economy. In Canada, with a very large proportion of our electricity generated from hydro and nuclear power, we can lead the world by showcasing the new sustainable electricity economy.
Much of the electricity generated in Canada now comes from sustainable hydropower, particularly in the provinces of Quebec, Manitoba and British Columbia. And there are still large untapped water resources available for sustainable electricity generation in these provinces. In B.C., for example, there are enough potential large-scale hydro projects to at least double current hydroelectric capacity on rivers without major impacts on fish habitat. There are also many possibilities for smaller scale run-of-the-river power projects that may have a smaller environmental impact.
Wind power resources are widely distributed across the country and wind is now starting to make small contributions to renewable electricity generation. Although wind power is currently the most economic renewable electricity source after hydro power, a wind turbine only generates electricity at its full rated capacity over a limited period of time. This low capacity factor results from the intermittent nature of wind resources and leads to a high capital cost per unit of energy generated. The intermittency of wind power also means that back-up energy storage is required in stand-alone applications, further adding to the overall capital cost. In Canada, with most of the population living far away from the best wind resources, the cost of building new electrical transmission facilities is also a major factor in making wind power expensive compared to our traditional hydro power and fossil fuel sources. However, as in many other countries, wind power installations are being encouraged using “feed-in tariffs,” a marketing term for subsidies.
The high capital cost of solar electricity facilities, both in the form of photovoltaic cells and large–scale solar thermal plants, means that these plants are unlikely to become commonplace in Canada for utility-scale generation in the near future. There is a role, however, for smaller scale distributed generation of electricity by photovoltaic cells, and for solar thermal generation of domestic hot water. Nuclear power is also well established in Canada, although in common with many other countries no new plants have been built for many years. There is a resurgence of interest in nuclear power around the world, however, and many countries that formerly had a moratorium on new nuclear plants, such as the UK, are now actively reconsidering this position. With our long history of building unique CANDU nuclear plants, it remains to be seen whether or not Canada will expand the role of nuclear power in a bid to reduce greenhouse gas emissions.
The successful development and introduction into the marketplace of grid-connected or plug-in hybrid vehicles will mark the beginning of a transition to the new electricity economy, eliminating the need for most passenger vehicles to use hydrocarbon fuels, at least for the majority of miles travelled. Electrically powered heat pumps to heat (and cool) our homes are the other crucial piece of the puzzle. If we ensure that new electricity generation facilities make use of only non-fossil energy resources, then we will be on the path to a more sustainable future. We know what needs to be done to preserve the planet for our children and grandchildren, and we need to let our elected leaders know that we know. In the words of my alma mater’s motto, Tuum est—it’s up to you!
Robert Evans is the author of Fueling Our Future: An Introduction to Sustainable Energy, published by Cambridge University Press in 2007 and short-listed for the 2008 Donner Prize. He is a professor of mechanical engineering at the University of British Columbia.